An immense chunk of ice detached from a glacier in the southwest of Greenland’s vast ice sheet during the autumn of 1911. In the subsequent months, it progressively melted as it was carried southward by ocean currents and the wind.
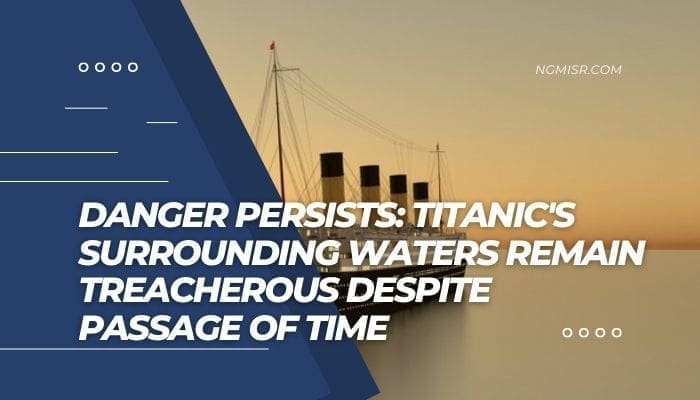
Then, on the cold, moonless night of April 14, 1912, a 125m-long (410ft) iceberg – all that remained of an estimated 500m (1,640ft) chunk of ice that had left a Greenland fjord the previous year – collided with the passenger ship RMS Titanic as it made its maiden voyage from Southampton, United Kingdom, to New York, United States. In less than three hours, more than 1,500 passengers and personnel perished when the ship sank. Approximately 400 miles (640 kilometers) southeast of the Newfoundland coast, the shipwreck now lies approximately 3.8 kilometers (12,500 feet) below the waters.
Icebergs continue to pose a threat to shipping; from March to August of 2019, 1,515 icebergs drifted far enough south to enter transatlantic shipping lanes. However, the Titanic’s final resting place poses its own dangers, making visits to the world’s most renowned shipwreck a formidable challenge.
Navigating the Depths
The deep ocean is pitch black. Sunlight cannot penetrate deeper than about 1,000 meters (3,300 feet) below the surface of water due to its rapid absorption. Beyond this point, there is perpetual darkness in the ocean. The Titanic is located within a region referred to as the “midnight zone” for this reason.
Previous expeditions to the wreck site have described descending through total darkness for more than two hours before the ocean floor abruptly appears beneath the submersible’s lights.
Due to the limited visibility beyond the few meters illuminated by the truck-sized submersible’s onboard lighting, navigating at this depth is difficult and it is easy to become disoriented on the seafloor.
Detailed maps of the Titanic disaster site created by decades of high-resolution scanning can provide waypoints as objects become visible. Sonar also enables the crew to detect features and objects beyond the submersible’s tiny illumination pool.
Pilots of submersibles also rely on a technique known as inertial navigation, which employs a system of accelerometers and gyroscopes to monitor their position and orientation relative to a known starting point and velocity. The Titan submersible from OceanGate is equipped with a state-of-the-art self-contained inertial navigation system, which it combines with a Doppler Velocity Log acoustic sensor to estimate the depth and speed of the vehicle relative to the sea floor.
However, passengers on previous OceanGate trips to the Titanic have described how difficult it is to locate their way upon reaching the ocean floor. Mike Reiss, a writer for The Simpsons who participated in a voyage to the Titanic with OceanGate last year, told the BBC, “When you reach the bottom, you don’t really know where you are. The Titanic is somewhere at the bottom of the ocean, but it was so dark that the largest object was only 500 yards (1,500 feet) distant, and we spent 90 minutes searching for it.
Desolate Depths
As an object descends deeper into the ocean, the surrounding water pressure increases. On the seafloor 3,800 meters (12,500 feet) below the surface, the Titanic and everything else is subjected to pressures approximately 40MPa, which are 390 times greater than those at the surface.
Robert Blasiak, an ocean researcher at the Stockholm Resilience Centre at Stockholm University, told BBC Radio 4’s Today program that this pressure is roughly 200 times greater than that of a vehicle tire. Therefore, you need a submersible with extremely substantial walls.
The carbon fiber and titanium walls of the Titan submersible are designed to allow it to operate at a maximum depth of 4,000 meters (13,123 feet).
Currents
We are presumably more familiar with the strong surface currents that can carry boats and swimmers off course, but the deep ocean is also swept by underwater currents. Although typically weaker than those at the surface, these can still entail the movement of substantial quantities of water. They can be driven by winds at the surface that affect the water column below, deep water tides, or thermohaline currents, which are caused by differences in water density due to temperature and salinity. Rare occurrences known as benthic storms, which are typically associated with surface eddies, can also produce powerful, sporadic currents that can remove material from the seafloor.
Information about the underwater currents surrounding the bow and stern portions of the Titanic, which broke apart as the ship sank, comes from studies of patterns in the seabed and the movement of squid around the wreck.
A portion of the Titanic wreck is located near to a section of seafloor affected by the Western Boundary Undercurrent, a stream of cold, southward-flowing water. This “bottom current” generates migratory dunes, ripples, and ribbon-shaped patterns in the sediment and silt along the ocean floor, which provide scientists with information about its strength. Most of the seafloor formations they’ve observed are associated with mild to moderate currents.
Sand ripples along the eastern edge of the Titanic debris field – the splatter of belongings, fittings, fixtures, coal, and parts of the ship that spread out as the ship sank – indicate an easterly to westerly bottom-flowing current, while within the main wreckage site, scientists say the currents trend from northwest to southwest, possibly because the larger pieces of the wreck alter their direction.
South of the bow section, the currents appear to be especially variable, shifting from northeast to northwest to southwest.
Numerous experts anticipate that the Titanic’s wreckage will be buried in sediment as a result of these currents.
Gerhard Seiffert, a deep-water marine archaeologist who recently led an expedition to scan the Titanic wreck in high resolution, told the BBC that he did not believe the area’s currents were powerful enough to pose a threat to a submersible, so long as it had sufficient power.
“I am unaware of any currents at the Titanic site that pose a threat to functioning deep-sea vehicles,” he says. “In the context of our mapping project, the currents represented a challenge for precision mapping, not a safety risk.”
The Collision Itself
After more than a century on the ocean floor, the Titanic has degraded progressively. Large portions of the wreckage were twisted and deformed by the initial collision of the two main sections of the ship when it collided with the seafloor. Microbes feeding on the ship’s iron have produced icicle-shaped “rusticles” and accelerated the ship’s deterioration over time. In fact, scientists estimate that the stern section of the ship is deteriorating 40 years faster than the bow section due to the higher bacterial activity there, which is primarily caused by the greater level of damage it sustained.
“The wreck is constantly collapsing, mainly due to corrosion,” says Seiffert. “Each year a small amount. However, as long as you maintain a secure distance — no direct contact, no penetration through openings — no harm is anticipated.”
Debris Travels
In the past, abrupt flows of sediment along the seabed have been known to damage and even remove man-made objects from the ocean floor, despite the fact that this is extremely unlikely.
Seismic events such as earthquakes initiate the largest of these events, such as the one that severed transatlantic cables off the coast of Newfoundland in 1929. There is a growing awareness of the danger posed by these events, although there is no evidence that a similar event played a role in the Titan submarine’s disappearance.
Over the years, scientists have discovered indications that the seafloor surrounding the Titanic wreck has been struck by massive underwater landslides in the remote past. Huge volumes of sediment appear to have cascaded down Newfoundland’s continental slope to form what scientists refer to as a “instability corridor.” They estimate that the last of these “destructive” events occurred tens of thousands of years ago, leaving behind sedimentary strata as thick as 100 meters (328 feet). David Piper, a marine geology research scientist with the Geological Survey of Canada who has spent many years studying the seabed around the Titanic, notes that such events are exceedingly rare. In terms of frequency, he compares such events to the eruption of Mount Vesuvius or Mount Fuji, which may occur every tens of thousands to hundreds of thousands of years.
Storms may initiate more frequent occurrences known as turbidity currents, which occur when water becomes laden with sediment and flows down the continental slope. Piper states, “We demonstrate a repeat interval of approximately 500 years.” However, the topography of the seafloor in the region would likely direct any sediment flows down “Titanic Valley,” preventing them from reaching the shipwreck.
Seiffert and Piper concur that it is unlikely that such an incident played a role in the Titan submersible’s disappearance.
Other geological features surrounding the wreck site have yet to be investigated. Paul-Henry Nargeolet, a former French Navy diver and submersible pilot, examined a mysterious blip detected by sonar in 1996 during a previous expedition to the Titanic with OceanGate. It turned out to be a rugged reef abundant with marine life. In his most recent expeditions, he had hoped to visit another blip he had detected near the Titanic ruins.
As the search for the Titan and its crew continues, there are few hints as to what may have happened to them. But in such a challenging and inhospitable environment, the risks of visiting the Titanic wreck are as pertinent today as they were in 1986, when the first individuals to see the shipwreck since it sank made the journey to the depths.